Advanced Earthquake Resistant Design Techniques |
|
Introduction
The conventional approach to earthquake resistant design of buildings depends upon providing the building with strength, stiffness and inelastic deformation capacity which are great enough to withstand a given level of earthquake–generated force. This is generally accomplished through the selection of an appropriate structural configuration and the careful detailing of structural members, such as beams and columns, and the connections between them.
In contrast, we can say that the basic approach underlying more advanced techniques for earthquake resistance is not to strengthen the building, but to reduce the earthquake–generated forces acting upon it. Among the most important advanced techniques of earthquake resistant design and construction are base isolation and energy dissipation devices.
|
|
Base Isolation
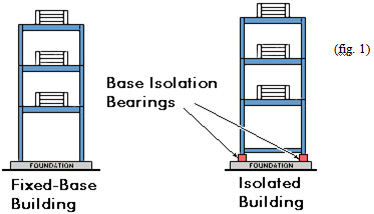
It is easiest to see this principle at work by referring directly to the most widely used of these advanced techniques, which is known as base isolation. A base isolated structure is supported by a series of bearing pads which are placed between the building and the building's foundation.(See Figure 1) A variety of different types of base isolation bearing pads have now been developed. For our example, we'll discuss lead–rubber bearings. These are among the frequently–used types of base isolation bearings. (See Figure 2) A lead–rubber bearing is made from layers of rubber sandwiched together with layers of steel. In the middle of the bearing is a solid lead 'plug.' On top and bottom, the bearing is fitted with steel plates which are used to attach the bearing to the building and foundation. The bearing is very stiff and strong in the vertical direction, but flexible in the horizontal direction.
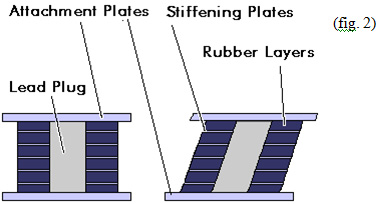
|
|
Earthquake Generated Forces
To get a basic idea of how base isolation works, first examine Figure 3. This shows an earthquake acting on both a base isolated building and a conventional, fixed–base, building. As a result of an earthquake, the ground beneath each building begins to move. In Figure 3, it is shown moving to the left.
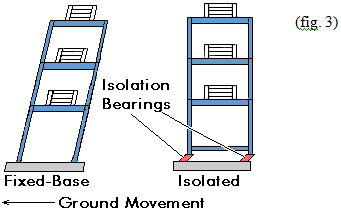
Each building responds with movement which tends toward the right. We say that the building undergoes displacement towards the right. The building's displacement in the direction opposite the ground motion is actually due to inertia. The inertial forces acting on a building are the most important of all those generated during an earthquake.
It is important to know that the inertial forces which the building undergoes are proportional to the building's acceleration during ground motion. It is also important to realize that buildings don't actually shift in only one direction.
Because of the complex nature of earthquake ground motion, the building actually tends to vibrate back and forth in varying directions. So, Figure 3 is really a kind of "snapshot" of the building at only one particular point of its earthquake response.
In addition to displacing toward the right, the un–isolated building is also shown to be changing its shape– from a rectangle to a parallelogram. We say that the building is deforming. The primary cause of earthquake damage to buildings is the deformation which the building undergoes as a result of the inertial forces acting upon it.
The different types of damage which buildings can suffer are quite varied and depend upon a large number of complicated factors. But to take one simple example, one can easily imagine what happens to two pieces of wood joined at a right angle by a few nails, when the very heavy building containing them suddenly starts to move very quickly — the nails pull out and the connection fails. |
|
Response of Base Isolated Building
By contrast, even though it too is displacing, the base–isolated building retains its original, rectangular shape. It is the lead–rubber bearings supporting the building that are deformed. The base–isolated building itself escapes the deformation and damage—which implies that the inertial forces acting on the base–isolated building have been reduced.
Experiments and observations of base–isolated buildings in earthquakes have been shown to reduce building accelerations to as little as 1/4 of the acceleration of comparable fixed–base buildings, which each building undergoes as a percentage of gravity. As we noted above, inertial forces increase, and decrease, proportionally as acceleration increases or decreases.
Acceleration is decreased because the base isolation system lengthens a building's period of vibration, the time it takes for the building to rock back and forth and then back again. And in general, structures with longer periods of vibration tend to reduce acceleration, while those with shorter periods tend to increase or amplify acceleration.
Finally, since they are highly elastic, the rubber isolation bearings don't suffer any damage. But what about that lead plug in the middle of our example bearing? It experiences the same deformation as the rubber. However, it also generates heat as it does so.
In other words, the lead plug reduces, or dissipates, the energy of motion—i.e., kinetic energy—by converting that energy into heat. And by reducing the energy entering the building, it helps to slow and eventually stop the building's vibrations sooner than would otherwise be the case —in other words, it damps the building's vibrations. (Damping is the fundamental property of all vibrating bodies which tends to absorb the body's energy of motion, and thus reduce the amplitude of vibrations until the body's motion eventually ceases.) |
|
Spherical Sliding Isolation Systems
As we said earlier, lead–rubber bearings are just one of a number of different types of base isolation bearings which have now been developed. Spherical Sliding Isolation Systems are another type of base isolation. The building is supported by bearing pads that have a curved surface and low friction.
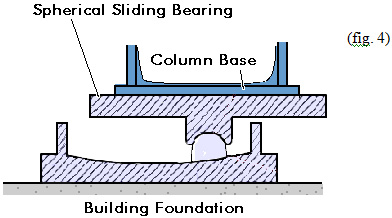
During an earthquake, the building is free to slide on the bearings. Since the bearings have a curved surface, the building slides both horizontally and vertically (See Figure 4.) The force needed to move the building upwards limits the horizontal or lateral forces which would otherwise cause building deformations. Also, by adjusting the radius of the bearing's curved surface, this property can be used to design bearings that also lengthen the building's period of vibration.
For more information read this article titled Protective Systems for Buildings: Application of Spherical Sliding Isolation Systems as it describes one particular type of spherical sliding isolation system, and its successful use in making some structures more earthquake resistant. |
|
Energy Dissipation Devices
The second of the major new techniques for improving the earthquake resistance of buildings also relies upon damping and energy dissipation, but it greatly extends the damping and energy dissipation provided by lead–rubber bearings.
As we've said, a certain amount of vibration energy is transferred to the building by earthquake ground motion. Buildings themselves do possess an inherent ability to dissipate, or damp, this energy. However, the capacity of buildings to dissipate energy before they begin to suffer deformation and damage is quite limited.
The building will dissipate energy either by undergoing large scale movement or sustaining increased internal strains in elements such as the building's columns and beams. Both of these eventually result in varying degrees of damage. So, by equipping a building with additional devices which have high damping capacity, we can greatly decrease the seismic energy entering the building, and thus decrease building damage.
Accordingly, a wide range of energy dissipation devices have been developed and are now being installed in real buildings. Energy dissipation devices are also often called damping devices. The large number of damping devices that have been developed can be grouped into three broad categories:
- Friction Dampers– these utilize frictional forces to dissipate energy
- Metallic Dampers– utilize the deformation of metal elements within the damper
- Viscoelastic Dampers– utilize the controlled shearing of solids
- Viscous Dampers– utilized the forced movement (orificing) of fluids within the damper
|
|
Fluid Viscous Dampers
Once again, to try to illustrate some of the general principles of damping devices, we'll look more closely at one particular type of damping device, the Fluid Viscous Damper, which is one variety of viscous damper that has been widely utilized and has proven to be very effective in a wide range of applications.
Damping Devices and Bracing Systems
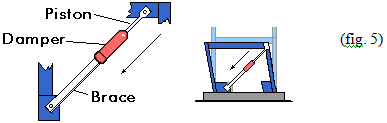
Damping devices are usually installed as part of bracing systems. Figure 5 shows one type of damper–brace arrangement, with one end attached to a column and one end attached to a floor beam. Primarily, this arrangement provides the column with additional support.
Most earthquake ground motion is in a horizontal direction; so, it is a building's columns which normally undergo the most displacement relative to the motion of the ground. Figure 5 also shows the damping device installed as part of the bracing system and gives some idea of its action.
|
Click here
for contact information.
|